What are the advantages & drawbacks of small angle X-ray scattering (SAXS) and transmission electron microscopy (TEM), and how do they complement each other?
The field of nanotechnology has seen a steady increase in interest from both public research institutions and commercial companies over the last two decades [1, 2]. Nowadays nanomaterials find applications in a plethora of sectors from biomedicine to microelectronics, food science and agriculture. These materials exhibit different electronic, optical, mechanical and chemical properties from their bulk counterparts [3]. Thus, comprehensive, accurate and reliable nanoscale level characterization plays a crucial role in the understanding and development of these materials. However, due to the inherent difficulties encountered in studying nanomaterials, it is oftentimes necessary to combine two or more characterization methods to obtain a full picture of the variety of properties associated with a particular system. Therefore, in order to choose and combine effectively the right methods, it is important to understand the strengths and limitations of the different techniques. Here we focus on presenting the roles of small angle X-ray scattering (SAXS) and transmission electron microscopy (TEM) in a comparative way with the aim to understand their unique capabilities as well as their complementarities.
SAXS
TEM
However, once the right sample is in the beam, TEM is able to produce some of the highest resolution structural analysis and eventually also provide elemental information. One of its strengths is that it offers a straightforward characterization of the shape and estimate of the particle size as well as a relative estimation of the samples’ degree of homogeneity/size dispersion. Accurate determination of particle parameters, such as size distribution, is complicated by a number of operator bias artifacts: as TEM is a local probe the choice of the analyzed section of the sample can be nonrepresentative of the entire material, the experimenter-guided inspection of single particles is time-consuming, limited to a few hundreds of particles and could favor the selection of larger particles which in general provide higher contrast, and finally, inhomogeneities can be formed during sample preparation (especially while removing the suspension liquid after dropcasting) [6]. Specific orientation of particle can be also induced at this preparation step generating wrong interpretation scenarios. Indeed, the image being formed by the projection of the object either by density contrast or phase contrast, the contribution of the 3D shape to the overall size might not be reachable except in the case of very anisotropic objects (such as discs) where an obvious orientation is observed (side of disc). Furthermore, imaging artifacts can be misleading, e.g., a population of discs can be falsely interpreted as bi population of discs and rods when it can be simply composed of a single population of discs with some of them being observed on their edge.
Therefore, depending on the goal of your research and its context, you might find that one of the techniques is sufficient to answer your questions. However, as SAXS and TEM offer complementary information, an exhaustive description of the sample properties, such as the size and shape of nanoparticles and their spatial distribution, or degree of homogeneity, can be obtained by combining the two methods. The modelization of SAXS can be done by an appropriate initialization using real space information of TEM and thus efficiently obtaining statistical data interpretation of the sample while maintaining it in natural conditions.
Particle size distribution of bimodal silica nanoparticles as revealed by SAXS and TEM
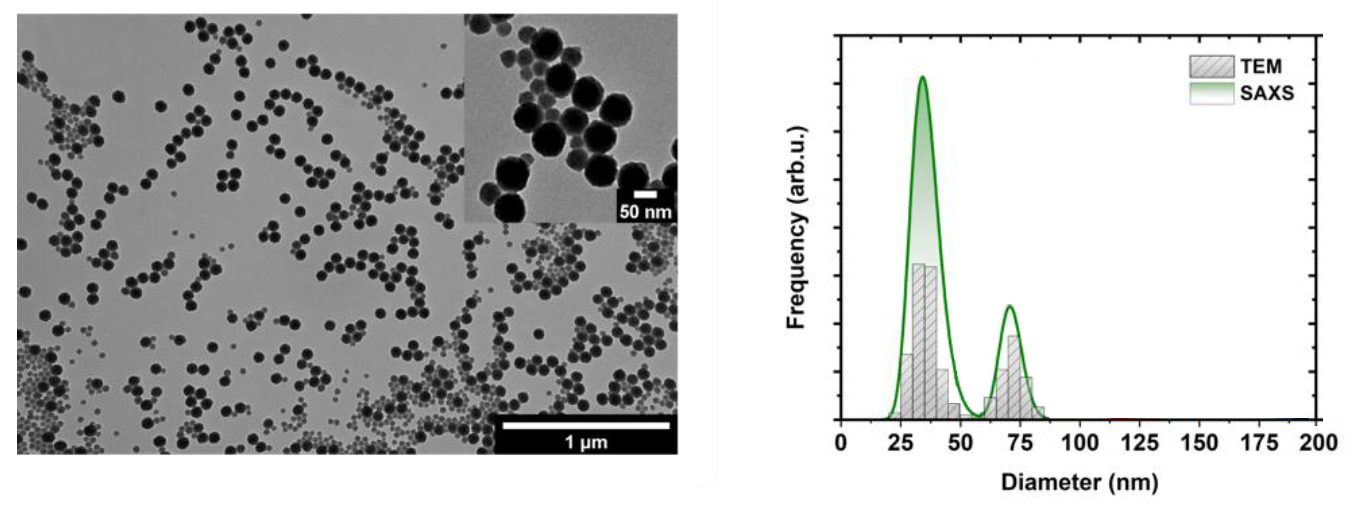
In conclusion, small angle x-ray scattering is a powerful routine characterization method for nanomaterials that benefits greatly from the use of microscopy techniques such as transmission electron microscopy to confirm the validity of the models used in the fitting routines.
SAXS/WAXS GISAXS | TEM | |
Types of samples | gel, solid, powder, liquid, turbid | Thin sections (< 100 nm) |
Sample preparation | Minimal | Intensive |
Volume probed | 0.1 to 1 mm^3 | Very small, thin |
Range of size | < 1 – 350 nm (up to 2.5 µm [PP1] USAXS) | < 1 nm – 1000 nm |
In-situ or operando | Yes | Yes |
Measuring time | Fast | Slow |
Statistics | Statistically relevant results | Poor |
Spatial Resolution | Low | High |
Data | Scattering pattern in reciprocal space | Image in real space |
Beam damage | No | Yes |
Limitations | Requires sufficient electron density contrast | Requires electrical conduction and high vacuum operation |
Information | Size, shape, crystallinity, orientation, bulk nanostructures and surface analysis capability in grazing mode | Morphology based on electron density and elemental chemical contrast and possibility to use diffraction contrast |
Source: Xenocs
Reference:
[1] Miyazaki, Kumiko, and Nazrul Islam. “Nanotechnology systems of innovation—An analysis of industry and academia research activities.” Technovation 27.11 (2007): 661-675.
[2] Woolley, Jennifer L., and Nydia MacGregor. “Science, technology, and innovation policy timing and nanotechnology entrepreneurship and innovation.” Plos one 17.3 (2022): e0264856.
[3] Mourdikoudis, Stefanos, Roger M. Pallares, and Nguyen TK Thanh. “Characterization techniques for nanoparticles: comparison and complementarity upon studying nanoparticle properties.” Nanoscale 10.27 (2018): 12871-12934.
[4] Hettler, Simon, Manuel Dries, Peter Hermann, Martin Obermair, Dagmar Gerthsen, and Marek Malac. “Carbon contamination in scanning transmission electron microscopy and its impact on phase-plate applications.” Micron 96 (2017): 38-47.
[5] Gleichmann, Nicole. “SEM vs TEM.” Technology Networks: Analysis & Separations, online (2020): https://www. technologynetwo rks. com/analysis/articles/sem-vs-tem-331262.
[6] Yang, Ye, Suiyang Liao, Zhi Luo, Runzhang Qi, Niamh Mac Fhionnlaoich, Francesco Stellacci, and Stefan Guldin. “Comparative characterisation of non-monodisperse gold nanoparticle populations by X-ray scattering and electron microscopy.” Nanoscale 12.22 (2020): 12007-12013.
[7] Al-Khafaji, Mohammed A., Anikó Gaál, András Wacha, Attila Bóta, and Zoltán Varga. “Particle size distribution of bimodal silica nanoparticles: A comparison of different measurement techniques.” Materials 13.14 (2020): 3101.